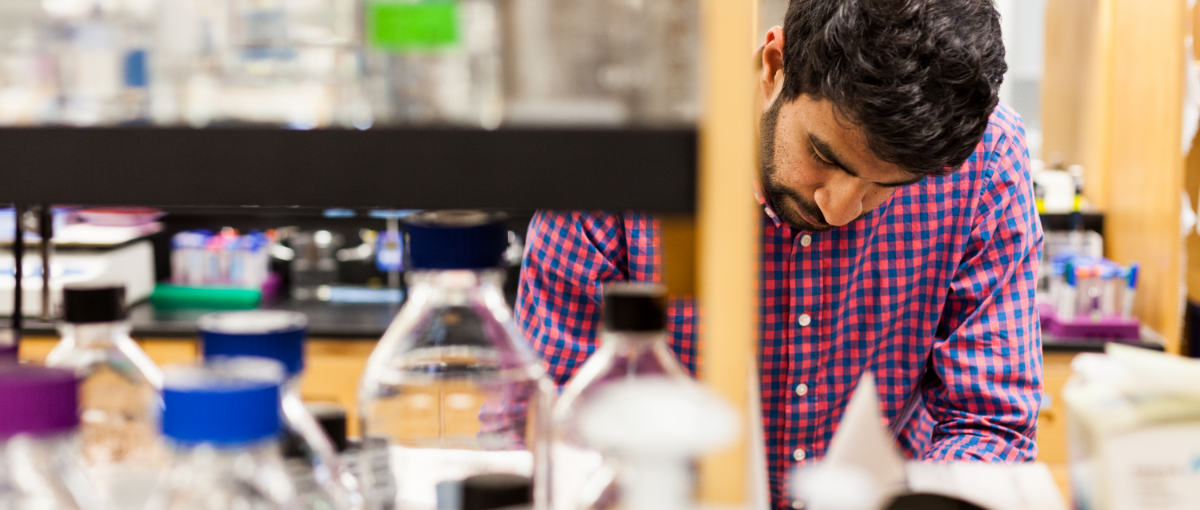
Proteins and enzymes play a central role in disease. We strive to uncover how they participate in antibiotic drug resistance, viral immune evasion and human genetic disorders.
Our viral immune evasion research
Unlike plants, animals and bacteria, which use separate genes to encode each of their required proteins, many viruses express long polyproteins from large ‘genes’, or open reading frames, that are subsequently cleaved into multiple functional protein units. The cleavage events are carried out by proteases that are found within the polyproteins themselves. The cleavages are essential to the virus life cycle making viral proteases excellent antiviral drug targets.
Currently, we focus on proteases from RNA viruses from the order Nidovirales, which contains viruses of animal and human importance, including arteriviruses, such as equine arteritis virus (EAV) and porcine reproductive and respiratory syndrome virus (PRRSV), and the severe acute respiratory syndrome (SARS) and emerging Middle-East respiratory syndrome (MERS) coronaviruses. These viruses express two polyproteins that contain multiple proteases that cleave them into function units. Remarkably, in addition to cleaving sites in the viral polyproteins, the one of the proteases removes the molecules ubiquitin (Ub) and ISG15 from cellular proteins to suppress antiviral pathways. Another of these proteases also assists virus replication by participating in ribosomal frameshifting events that yield additional viral proteins during virus genome translation.
Using molecular and structural biology methods, we explore the molecular basis underlying the multifunctional role of these proteases in the replication and host immune evasion strategies of viruses. This research area of our lab has yielded important new insights into the central role proteases play in in virus replication and immune evasion.
Our Antibiotic resistance research
Antibiotic resistance is a growing problem of major societal concern. If left unchecked, it is estimated that by 2050 antibiotic resistant infections will kill more people annually than cancer according to a 2014 study published by the UK government. The mainstay treatment for bacterial infections are beta-lactam antibiotics, which constitute over 50% of all antibiotics in clinical use. However, their efficacy is being eroded by the increasing prevalence of bacterial resistance mechanisms and new strategies are urgently needed to sustain the efficacy of this safe and proven antibiotic class.
We found that the bacterial glycosidase NagZ can be targeted to suppress beta-lactam antibiotic resistance in Pseudomonas aeruginosa, a bacterium that is the leading cause of lung infections and mortality among cystic fibrosis patients, as well as being a major cause of severe hospital-acquired infections. The NagZ enzyme is involved in the peptidoglycan recycling pathway of Gram-negative bacteria, such Pseudomonas aeruginosa, and this pathway regulates the expression of AmpC beta-lactamase, an enzyme that inactivates most clinically available beta-lactams. P. aeruginosa and a number of other bacterial pathogens produces AmpC when challenged with beta-lactam antibiotics, making them highly resistant to this safe and proven class of antibiotics. We are engaged in fully understanding the biochemical pathway that allows these bacteria induce production of AmpC in response to beta-lactam treatment and use this knowledge to guide new therapeutic approaches to block this mechanism of antibiotic resistance.
Our genetic disease research
Tay-Sachs disease and Sandhoff Disease (also knows as the GM2 gangliosidoses) are inherited neurodegenerative diseases that result from genetic mutations that inactivate an enzyme known as HexA. HexA is a protein in our cells that degrades GM2-ganglioside (GM2), a molecule found primarily on neurons of the central and peripheral nervous system. Loss of HexA activity results in rapid accumulation of GM2 in neurons and severe neurological deterioration. The rate of GM2 accumulation depends on how severely the genetic mutations impact HexA activity. Totally inactive HexA leads to rapid GM2 accumulation and death by 1-4 years of age; whereas, mutations that allow for some residual HexA activity (1-10% of normal), GM2 accumulation is slowed and disease progression is delayed. While still neurologically debilitating, these late-onset forms of the disease may not significantly impact lifespan.
Importantly, HexA activity as low as 10% can prevent, and possibly reverse, GM2 accumulation and the associated neurodegeneration. We hypothesize that a therapy for infantile and late-onset forms of GM2 gangliosidosis is achievable by supplementing patients with active Hex enzyme (i.e. enzyme replacement therapy), and in late-onset cases, combing enzyme replacement with drugs known to boost residual Hex activity to bring the total Hex activity above the critical 10% activity threshold. We have recently engineered HexA to markedly improve its stability and function for enzyme replacement therapy. We now propose to use the engineered enzyme, known as HexM, to develop an enzyme replacement therapy that will be evaluated in mouse models of GM2 gangliosidosis in combination with a drug known to rescue residual HexA activity in cells. Our approach holds promise to reduce GM2 accumulation and reverse the progression of this debilitating family of neurological diseases.
